"The hurrier I go the behinder I get."
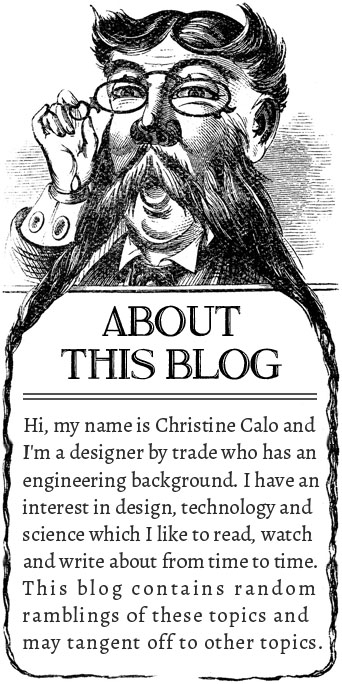
Search This Blog
Categories
Featured Post
The Infinite Game - by Simon Sinek
‘The Infinite Game’ tries to answer the question ‘How do we win a game that has no end?’ The author Simon Sinek describes 2 types of games. ...
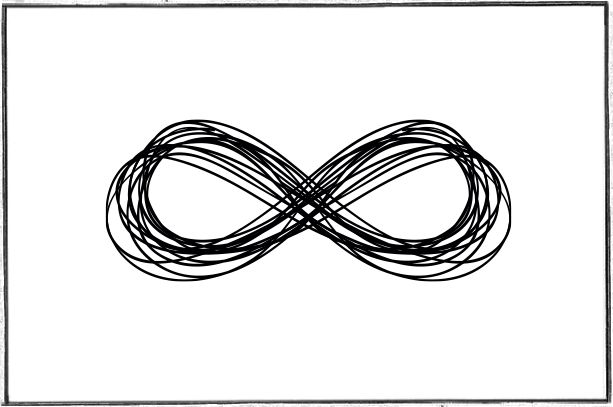
Past Entries
-
▼
2021
(
20
)
-
▼
August
(
9
)
- Bounce Back - by Susan Kahn
- The Selfish Gene - by Richard Dawkins
- Scale - by Geoffrey West
- The Evolution of Everything - by Matt Ridley
- The Inevitable - by Kevin Kelly
- The Attention Merchants - by Tim Wu
- Sizing People Up - by Robin Dreeke & Cameron Stauth
- Atomic Habits - by James Clear
- Zero to One - by Peter Thiel
-
▼
August
(
9
)